Comparison of mean whole blood and mean platelet-rich plasma (PRP) platelet concentrations between 5 groups. An asterisk (*) indicates a statistically significant difference between the mean whole blood and mean PRP product for that group. Bars represent mean ± SD (the number of syringes for groups 1 through 5 was 10, 11, 12, 12, and 11, respectively).
Comparison of ratios between PRP platelet concentration and whole blood platelet concentrations. A platelet fold change of 1 means that those 2 concentrations are equal. The mean of groups 1, 3, 4, and 5 fall above this line. Box and whisker plots represent mean ± 95% CI, with dots representing outliers. Medical Ultrasound Instruments
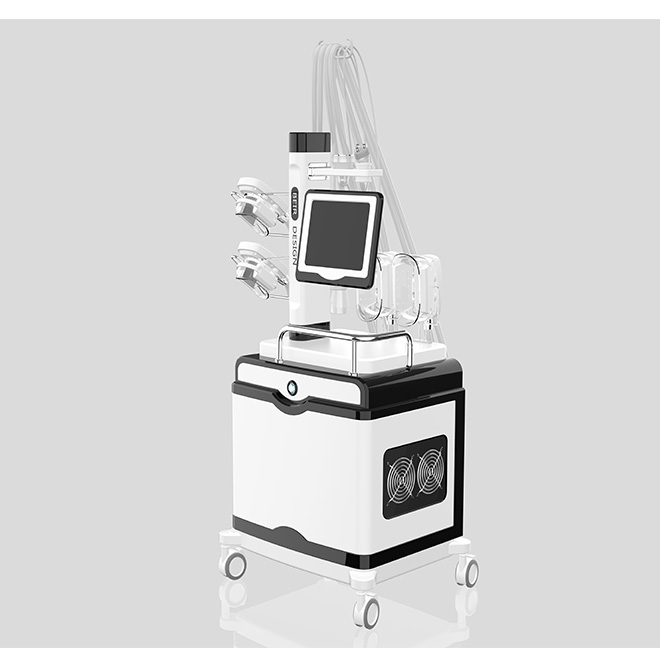
Comparison of mean whole blood and mean platelet-rich plasma (PRP) platelet concentrations between 5 groups. An asterisk (*) indicates a statistically significant difference between the mean whole blood and mean PRP product for that group. Bars represent mean ± SD (the number of syringes for groups 1 through 5 was 10, 11, 12, 12, and 11, respectively).
Comparison of ratios between PRP platelet concentration and whole blood platelet concentrations. A platelet fold change of 1 means that those 2 concentrations are equal. The mean of groups 1, 3, 4, and 5 fall above this line. Box and whisker plots represent mean ± 95% CI, with dots representing outliers.
Alsousou J , Thompson M , Hulley P , Noble A , Willett K . The biology of platelet-rich plasma and its application in trauma and orthopaedic surgery: a review of the literature . J Bone Joint Surg Br . 2009 ; 91 ( 8 ): 987 - 996 . doi: 10.1302/0301-620X.91B8.22546
Garbin LC , Olver CS . Platelet-rich products and their application to osteoarthritis . J Equine Vet Sci . 2020 ; 86 : 102820 . doi: 10.1016/j.jevs.2019.102820
Nguyen RT , Borg-Stein J , McInnis K . Applications of platelet-rich plasma in musculoskeletal and sports medicine: an evidence-based approach . PM R . 2011 ; 3 ( 3 ): 226 - 250 . doi: 10.1016/j.pmrj.2010.11.007
Frisbie DD , Kawcak CE , Werpy NM , Park RD , McIlwraith CW . Clinical, biochemical, and histologic effects of intra-articular administration of autologous conditioned serum in horses with experimentally induced osteoarthritis . Am J Vet Res . 2007 ; 68 ( 3 ): 290 - 296 . doi: 10.2460/ajvr.68.3.290
McCarrel TM , Mall NA , Lee AS , Cole BJ , Butty DC , Fortier LA . Considerations for the use of platelet-rich plasma in orthopedics . Sports Med . 2014 ; 44 ( 8 ): 1025 - 1036 . doi: 10.1007/s40279-014-0195-5
Marx RE . Platelet-rich plasma: evidence to support its use . J Oral Maxillofac Surg . 2004 ; 62 ( 4 ): 489 - 496 . doi: 10.1016/j.joms.2003.12.003
McCarrel TM , Minas T , Fortier LA . Optimization of leukocyte concentration in platelet-rich plasma for the treatment of tendinopathy . J Bone Joint Surg Am . 2012 ; 94 ( 19 ): e143 . doi: 10.2106/JBJS.L.00019
Brossi PM , Moreira JJ , Machado TSL , Baccarin RYA . Platelet-rich plasma in orthopedic therapy: a comparative systematic review of clinical and experimental data in equine and human musculoskeletal lesions . BMC Vet Res . 2015 ; 11 ( 1 ): 98 . doi: 10.1186/s12917-015-0403-z
Sharun K , Jambagi K , Dhama K , Kumar R , Pawde AM , Amarpal . Therapeutic potential of platelet-rich plasma in canine medicine . Arch Razi Inst . 2021 ; 76 ( 4 ): 721 - 730 . doi: 10.22092/ari.2021.355953.1749
King W , Cawood K , Bookmiller M . The use of autologous protein solution (Pro-Stride®) and leukocyte-rich platelet-rich plasma (Restigen®) in canine medicine . Vet Med (Auckl) . 2021 ; 12 : 53 - 65 . doi: 10.2147/VMRR.S286913
Edelmann ML , Mohammed HO , Wakshlag JJ , Ledbetter EC . Clinical trial of adjunctive autologous platelet-rich plasma treatment following diamond-burr debridement for spontaneous chronic corneal epithelial defects in dogs . J Am Vet Med Assoc . 2018 ; 253 ( 8 ): 1012 - 1021 . doi: 10.2460/javma.253.8.1012
Silva RF , Carmona JU , Rezende CMF . Intra-articular injections of autologous platelet concentrates in dogs with surgical reparation of cranial cruciate ligament rupture: a pilot study . Vet Comp Orthop Traumatol . 2013 ; 26 ( 4 ): 285 - 290 . doi: 10.3415/VCOT-12-06-0075
Fahie MA , Ortolano GA , Guercio V , et al. A randomized controlled trial of the efficacy of autologous platelet therapy for the treatment of osteoarthritis in dogs . J Am Vet Med Assoc . 2013 ; 243 ( 9 ): 1291 - 1297 . doi: 10.2460/javma.243.9.1291
Stief M , Gottschalk J , Ionita JC , Einspanier A , Oechtering G , Böttcher P . Concentration of platelets and growth factors in canine autologous conditioned plasma . Vet Comp Orthop Traumatol . 2011 ; 24 ( 2 ): 122 - 125 . doi: 10.3415/VCOT-10-04-0064
Perego R , Spada E , Baggiani L , Martino PA , Proverbio D . Efficacy of a semi automated commercial closed system for autologous leukocyte- and platelet-rich plasma (l-prp) production in dogs: a preliminary study . Animals (Basel) . 2020 ; 10 ( 8 ): 1342 . doi: 10.3390/ani10081342
DeMello V , Chen G , Wakshlag J , Mason D . Evaluation of platelet and leukocyte counts in canine platelet-rich plasma obtained after successive blood collections from the same patient and the effects of freezing on the concentration of growth factors present in it . Front Vet Sci . 2022 ; 9 : 838481 . doi: 10.3389/fvets.2022.838481
Franklin SP , Birdwhistell KE . Assessment of canine Autologous Conditioned PlasmaTM cellular and transforming growth factor-β1 content . Front Vet Sci . 2018 ; 5 : 105 . doi: 10.3389/fvets.2018.00105
Franklin SP , Burke EE , Holmes SP . The effect of platelet-rich plasma on osseous healing in dogs undergoing high tibial osteotomy . PLoS One . 2017 ; 12 ( 5 ): e0177597 . doi: 10.1371/journal.pone.0177597
Farghali HA , AbdElKader NA , AbuBakr HO , et al. Antimicrobial action of autologous platelet-rich plasma on MRSA-infected skin wounds in dogs . Sci Rep . 2019 ; 9 ( 1 ): 12722 . doi: 10.1038/s41598-019-48657-5
Sundman EA , Cole BJ , Fortier LA . Growth factor and catabolic cytokine concentrations are influenced by the cellular composition of platelet-rich plasma . Am J Sports Med . 2011 ; 39 ( 10 ): 2135 - 2140 . doi: 10.1177/0363546511417792
Frye CW , Enders A , Brooks MB , Struble AM , Wakshlag JJ . Assessment of canine autologous platelet-rich plasma produced with a commercial centrifugation and platelet recovery kit . Vet Comp Orthop Traumatol . 2016 ; 29 ( 1 ): 14 - 19 . doi: 10.3415/VCOT-15-03-0046
de Marcos Carpio I , Sanghani-Kerai A , Solano MA , Blunn G , Jifcovici A , Fitzpatrick N . Clinical cohort study in canine patients, to determine the average platelet and white blood cell number and its correlation with patient’s age, weight, breed and gender: 92 cases (2019-2020) . Vet Sci . 2021 ; 8 ( 11 ): 262 . doi: 10.3390/vetsci8110262
Cherno B , Alvarez L , Lamb KE , Canapp S . The Stryker Regenkit PRP Kit does not concentrate canine platelets . Front Vet Sci . 2020 ; 7 : 555909 . doi: 10.3389/fvets.2020.555909
Franklin SP , Garner BC , Cook JL . Characteristics of canine platelet-rich plasma prepared with five commercially available systems . Am J Vet Res . 2015 ; 76 ( 9 ): 822 - 827 . doi: 10.2460/ajvr.76.9.822
Boswell SG , Schnabel LV , Mohammed HO , Sundman EA , Minas T , Fortier LA . Increasing platelet concentrations in leukocyte-reduced platelet-rich plasma decrease collagen gene synthesis in tendons . Am J Sports Med . 2014 ; 42 ( 1 ): 42 - 49 . doi: 10.1177/0363546513507566
Carr BJ , Canapp SO Jr , Mason DR , Cox C , Hess T . Canine platelet-rich plasma systems: a prospective analysis . Front Vet Sci . 2016 ; 2 : 73 . doi: 10.3389/fvets.2015.00073
Shmalberg J . The Arthrex Autologous Conditioned Plasma Double Syringe System® for canine use . White paper. Arthrex Inc ; 2015 .
Dohan Ehrenfest DM , Rasmusson L , Albrektsson T . Classification of platelet concentrates: from pure platelet-rich plasma (P-PRP) to leucocyte- and platelet-rich fibrin (L-PRF) . Trends Biotechnol . 2009 ; 27 ( 3 ): 158 - 167 . doi: 10.1016/j.tibtech.2008.11.009
Clemmons RM , Bliss EL , Dorsey-Lee MR , Seachord CL , Meyers KM . Platelet function, size and yield in whole blood and in platelet-rich plasma prepared using differing centrifugation force and time in domestic and food-producing animals . Thromb Haemost . 1983 ; 50 ( 4 ): 838 - 843 . doi: 10.1055/s-0038-1665324
Oshinowo O , Copeland R , Sakurai Y , et al. Significant differences in single-platelet biophysics exist across species but attenuate during clot formation . Blood Adv . 2021 ; 5 ( 2 ): 432 - 437 . doi: 10.1182/bloodadvances.2020003755
Cook JL , Smith PA , Bozynski CC , et al. Multiple injections of leukoreduced platelet rich plasma reduce pain and functional impairment in a canine model of ACL and meniscal deficiency . J Orthop Res . 2015 ; 34 ( 4 ): 607 - 615 .
Bozynski CC , Stannard JP , Smith P , et al. Acute management of anterior cruciate ligament injuries using novel canine models . J Knee Surg . 2016 ; 29 ( 7 ): 594 - 603 . doi: 10.1055/s-0035-1570115
Lee AS , Ellman MB , Yan D , et al. A current review of molecular mechanisms regarding osteoarthritis and pain . Gene . 2013 ; 527 ( 2 ): 440 - 447 . doi: 10.1016/j.gene.2013.05.069
Akeda K , An HS , Okuma M , et al. Platelet-rich plasma stimulates porcine articular chondrocyte proliferation and matrix biosynthesis . Osteoarthritis Cartilage . 2006 ; 14 ( 12 ): 1272 - 1280 . doi: 10.1016/j.joca.2006.05.008
Diehl KH , Hull R , Morton D , et al. ; European Federation of Pharmaceutical Industries Association and European Centre for the Validation of Alternative Methods. A good practice guide to the administration of substances and removal of blood, including routes and volumes . J Appl Toxicol . 2001 ; 21 ( 1 ): 15 - 23 . doi: 10.1002/jat.727
Lee MI , Kim JH , Kwak HH , et al. A placebo-controlled study comparing the efficacy of intra-articular injections of hyaluronic acid and a novel hyaluronic acid-platelet-rich plasma conjugate in a canine model of osteoarthritis . J Orthop Surg Res . 2019 ; 14 ( 1 ): 314 . doi: 10.1186/s13018-019-1352-1
To evaluate the effect of variable centrifugation protocols on the cellular composition of the final product of a canine autologous conditioned plasma double-syringe system.
30 client-owned healthy adult medium- to large-breed (17- to 45-kg) dogs.
35 mL of anticoagulated whole blood from each subject was aliquoted into 3 samples: a baseline and 2 double syringes. The syringes were processed for platelet-rich plasma (PRP). Each double syringe was randomly assigned to 1 of 5 groups, which varied in centrifugation settings between 580 and 1,304 X g and 5 and 10 minutes. CBC analysis was performed on each of the samples to determine cellular composition. A mixed-effect linear model was fit to the data.
60 PRP samples and 30 whole blood samples were analyzed. Manufacturer settings generated a platelet fold change > 1 but did not increase concentration to the extent expected. When comparing speed alone, increased centrifugation force was associated with lower platelet fold change. When comparing time alone, increased centrifugation time was also associated with lower platelet fold change and lower leukocyte concentration.
Autologous conditioned plasma double syringes require a low volume of initial whole blood, making them preferable for canine PRP in clinical settings. This study aimed to evaluate the effect of the centrifugation protocol on the final product cellular composition in dogs and add to the available data on protocols to maximize platelet yield in PRP. Due to inherent individual variability, this study emphasized the importance of evaluating biological samples prior to administration to predict and improve patient outcomes.
To evaluate the effect of variable centrifugation protocols on the cellular composition of the final product of a canine autologous conditioned plasma double-syringe system.
30 client-owned healthy adult medium- to large-breed (17- to 45-kg) dogs.
35 mL of anticoagulated whole blood from each subject was aliquoted into 3 samples: a baseline and 2 double syringes. The syringes were processed for platelet-rich plasma (PRP). Each double syringe was randomly assigned to 1 of 5 groups, which varied in centrifugation settings between 580 and 1,304 X g and 5 and 10 minutes. CBC analysis was performed on each of the samples to determine cellular composition. A mixed-effect linear model was fit to the data.
60 PRP samples and 30 whole blood samples were analyzed. Manufacturer settings generated a platelet fold change > 1 but did not increase concentration to the extent expected. When comparing speed alone, increased centrifugation force was associated with lower platelet fold change. When comparing time alone, increased centrifugation time was also associated with lower platelet fold change and lower leukocyte concentration.
Autologous conditioned plasma double syringes require a low volume of initial whole blood, making them preferable for canine PRP in clinical settings. This study aimed to evaluate the effect of the centrifugation protocol on the final product cellular composition in dogs and add to the available data on protocols to maximize platelet yield in PRP. Due to inherent individual variability, this study emphasized the importance of evaluating biological samples prior to administration to predict and improve patient outcomes.
Platelet-rich plasma (PRP) continues to increase in popularity as a biologic regenerative therapy option and is applied to an ever-expanding array of conditions across numerous species.1,2 PRP originated in human medicine and was then adopted in veterinary medicine, initially with equine patients.3–8 It is now increasingly common in canine medicine for the treatment of soft tissue injuries, wound healing, bone healing, the treatment of tendon and ligament injuries, and patients experiencing clinical signs of osteoarthritis.9–13 The benefit of PRP administration is attributed to the high concentration of growth factors contained within the alpha granules released when platelets are activated. These alpha granules release a variety of growth factors, including but not limited to platelet-derived growth factor, insulin-like growth factor, transforming growth factor-β1 and β2, vascular endothelial growth factor, basic fibroblastic growth factor, and epidermal growth factor.14–16 These growth factors have been shown to mitigate inflammation, initiate anabolic processes, and regenerate tissue.17,18 In contrast, other components of PRP, particularly leukocytes, are thought to release proinflammatory cytokines that can exacerbate or perpetuate inflammation and potentially impede tissue healing.19 As shown by Sundman et al,20 growth factor and catabolic cytokine concentrations are influenced by the cellular composition of PRP. Therefore, the cell distribution created through the use of commercial PRP systems will likely ultimately impact patients’ clinical outcomes.
The therapeutic use of PRP has faced substantial criticism over the years due to gaps in the understanding of the best methods for generating a consistent, effective final product. The systems used to generate PRP vary by blood volume, use of anticoagulant, number of spins, and centrifugation speed, resulting in drastically different cell distributions.21,22 The variation in clinical outcomes is further compounded by administration variables such as dose volume, timing of treatment, and redosing intervals. Because of these variables, some studies have concluded that there is no clinical benefit of PRP.23 However, these conclusions can often be traced to the generation of PRP that is inconsistent in quality. The makeup of an ideal PRP product (concentration of platelets, neutrophils, macrophages, total leukocytes, and erythrocytes) remains unknown.24,25 Extensive clinical trials are necessary to determine the makeup of an ideal canine PRP product. This study examined a specific widely used commercial system to optimize variables in the canine patient for that specific system.
The objective of this study was to evaluate the cellular composition of the final product of a double-syringe system when subjected to different centrifugation speeds and times. This system requires 13.5 mL of whole blood, substantially less than any other system on the market, making it ideal for small-animal patients.26 It is also straightforward to use, with relatively short spin times (5 to 10 minutes), while still generating a clinically useful volume of PRP. Through quantifying the platelet concentrations and fold changes achieved and assessing leukocyte and erythrocyte concentrations or reductions in the PRP products, an optimal protocol for PRP generation with this system can be established. This study aimed to answer a necessary question along the path to generating consistent and effective PRP in canine patients. We hypothesized that manufacturer direction settings would produce PRP with platelet concentrations greater than that of whole blood. We further hypothesized that increasing centrifugation force and time would negatively impact the concentration of platelets.
This ex vivo experimental study was performed from July to December 2022 at a single private practice referral center. Thirty healthy adult dogs were recruited on a volunteer basis to participate in this study. These dogs were either employee-owned pets or patients of the sports medicine service undergoing elective orthopedic procedures. Owner consent for participation in the study was obtained. All animals were evaluated to be free of medical problems as determined by physical examination (performed by a veterinary surgical resident), clinical history, and CBC analysis. All procedures were directly overseen by a licensed veterinarian.
The system used in this study was the Arthrex autologous conditioned plasma (ACP) double-syringe system. The blood collection for PRP preparation was performed following the manufacturer’s instructions.27 The Arthrex system consists of a single centrifugation method using 13.5 mL of whole blood to produce PRP.
Thirty-one milliliters of whole blood was aseptically collected from the jugular or cephalic vein of each patient. The whole blood was aspirated into a 35-mL syringe, while rocking the syringe carefully to allow for mixing of the blood with 4 mL of anticoagulant citrate dextrose solution (Solution A; ACD-A). The anticoagulant-to-blood ratio was maintained from manufacturer directions. Whole blood was evaluated with a CBC analysis for baseline hematologic analysis (1 to 2 mL used/CBC analysis), and the remainder was processed for PRP.
Fifteen milliliters of the anticoagulated blood was transferred into 2 double syringes. Each double syringe was randomly assigned to 1 of 5 groups, which varied by centrifugation force and time (Table 1). These syringes were then placed into the centrifuge (Rotofix 32; Andreas Hettich GmbH and Co KG) with appropriate counterbalances, without the brake engaged, at the assigned speed and time. The group centrifugation speeds and times were based on those established at the authors’ institution.
Blood from each subject was fractioned into 3 aliquots: a baseline sample and 2 double syringes to be processed into platelet-rich plasma. Each double syringe was randomly assigned to 1 of the 5 groups, which varied in centrifugation speed and time.
After the 1-step centrifugation process, double syringes were removed, with care taken to keep them in the upright position so as to not disturb the plasma layer. The total volume of PRP generated was measured and recorded. PRP was then transferred into the inner syringe until the tip of the smaller syringe reached the transition layer and a flash was obtained. The smaller syringe was removed and agitated to distribute platelets uniformly in solution. An aliquot of each PRP was placed in a lavender-top (EDTA) tube and then routinely shipped with the whole blood sample to a commercial laboratory (IDEXX Laboratories Inc). The results were returned electronically within 24 to 48 hours of submission.
Baseline descriptive statistics were calculated for all measured variables. Linear mixed models were used to estimate differences from baseline by group, with a random effect of each dog to account for correlated observation from the same animal. Linear mixed models were also used to estimate the independent effects of speed and time on the final product. These models were run separately for each measured variable, and models in which the final value was the outcome were adjusted for the baseline value of that variable. P values (P < .005 considered significant) are presented unadjusted for multiple comparisons; the a priori primary comparison was the effect of speed and time on mean platelets in the final product.
A total of 90 CBCs were performed on 60 PRP and 30 peripheral whole blood samples. The average amount of PRP produced was 3.5 mL (range, 0.5 to 7.5 mL) per double syringe. Data from 2 dogs were excluded from the statistical analysis of platelet fold change due to initial thrombocytopenia (flagged in an automated CBC report and confirmed by a clinical pathologist), raising concern for the patient’s systemic health.
Blood was obtained from a total of 30 healthy adult dogs. The following breeds were represented: German Shepherd Dog (n = 6), Pit Bull–type breed (5), Husky or Husky mix (4), Labrador Retriever (3), Weimaraner (2), Border Collie (2), Boxer (1), Goldendoodle (1), Labradoodle (1), Australian Cattle Dog (1), English Bulldog mix (1), and mixed breed (3). The patients ranged from 1.5 to 12 years of age, with a median age of 5 years. The patients ranged from 17 to 45 kg, with a median weight of 24.75 kg. Sixteen of the study participants were neutered males, 13 were spayed females, and 1 was an intact female. The samples were randomly assigned to groups, and there were no significant differences between the demographics of cohorts in each group.
The only group with statistically significant platelet increase compared to whole blood baseline was group 3, which was prepared using a centrifugation speed of 580 X g for 5 minutes (Figure 1).
Comparison of mean whole blood and mean platelet-rich plasma (PRP) platelet concentrations between 5 groups. An asterisk (*) indicates a statistically significant difference between the mean whole blood and mean PRP product for that group. Bars represent mean ± SD (the number of syringes for groups 1 through 5 was 10, 11, 12, 12, and 11, respectively).
Citation: Journal of the American Veterinary Medical Association 2023; 10.2460/javma.23.04.0218
Comparison of mean whole blood and mean platelet-rich plasma (PRP) platelet concentrations between 5 groups. An asterisk (*) indicates a statistically significant difference between the mean whole blood and mean PRP product for that group. Bars represent mean ± SD (the number of syringes for groups 1 through 5 was 10, 11, 12, 12, and 11, respectively).
Citation: Journal of the American Veterinary Medical Association 2023; 10.2460/javma.23.04.0218
Comparison of mean whole blood and mean platelet-rich plasma (PRP) platelet concentrations between 5 groups. An asterisk (*) indicates a statistically significant difference between the mean whole blood and mean PRP product for that group. Bars represent mean ± SD (the number of syringes for groups 1 through 5 was 10, 11, 12, 12, and 11, respectively).
Citation: Journal of the American Veterinary Medical Association 2023; 10.2460/javma.23.04.0218
Seventy-five percent (9/12 syringes) of PRP generated in group 3 had a fold change > 10%. In contrast, only 18% (2/11 syringes) PRP samples in group 2, which was prepared using a centrifugation speed of 906 X g for 10 minutes, had a fold change > 10% (Figure 2).
Comparison of ratios between PRP platelet concentration and whole blood platelet concentrations. A platelet fold change of 1 means that those 2 concentrations are equal. The mean of groups 1, 3, 4, and 5 fall above this line. Box and whisker plots represent mean ± 95% CI, with dots representing outliers.
Citation: Journal of the American Veterinary Medical Association 2023; 10.2460/javma.23.04.0218
Comparison of ratios between PRP platelet concentration and whole blood platelet concentrations. A platelet fold change of 1 means that those 2 concentrations are equal. The mean of groups 1, 3, 4, and 5 fall above this line. Box and whisker plots represent mean ± 95% CI, with dots representing outliers.
Citation: Journal of the American Veterinary Medical Association 2023; 10.2460/javma.23.04.0218
Comparison of ratios between PRP platelet concentration and whole blood platelet concentrations. A platelet fold change of 1 means that those 2 concentrations are equal. The mean of groups 1, 3, 4, and 5 fall above this line. Box and whisker plots represent mean ± 95% CI, with dots representing outliers.
Citation: Journal of the American Veterinary Medical Association 2023; 10.2460/javma.23.04.0218
The RBC concentration was significantly decreased from baseline in all groups, though there was not a statistically significant difference among the groups. The overall leukocyte concentration was significantly decreased in all groups, except for group 3 (Table 2). Group 3 had a significantly decreased neutrophil concentration but significantly increased lymphocyte and monocyte concentrations, compared to baseline.
Comparison of leukocyte and Hct concentrations compared to baseline means by group, as shown by the difference from baseline and 95% CI.
When comparing the distribution of blood components at the 3 centrifugation forces (580, 906, and 1,304 X g), while holding the time variable constant, there was a statistically significant difference between platelet concentrations at the lowest speed compared to the 2 higher speeds (Table 3). There were no significant differences between total leukocyte, neutrophil, or monocyte concentrations among the rates. However, when compared to 1,304 X g, 580 X g did yield a significantly lower lymphocyte concentration. When comparing the distribution of blood components at the 2 centrifugation times (5 and 10 minutes), while holding the speed variable constant, there was no statistically significant difference between neutrophil concentrations. However, 5 minutes yielded a higher platelet concentration as well as higher total leukocyte, monocyte, and lymphocyte concentrations.
Comparison of blood cell concentrations between speed and time variables, as shown by the difference between the variables and 95% CI. Negative values indicate that the first value is lower than the second, while holding the other variable constant.
See Tables 1 and 2 for key.
The results of this study supported the acceptance of both hypotheses 1 and 2. The first hypothesis was that the manufacturer settings would generate a platelet fold change > 1, which was supported by an average result of 1.31 times that of baseline, with 50% of samples in group 1 generating a > 10% increase compared to the baseline platelet count. These results are lower than previously reported, with prior studies reporting a platelet fold change between 1.55 and 2.5 times that of baseline.27 The second hypothesis was that when comparing variables alone, increasing centrifugation speed or time would be associated with lower platelet fold change, which was supported by analysis of the individual variables. However, none of the protocols tested using the ACP double-syringe system generated final products with a platelet fold change consistent with the general definition of PRP, which has a fold change of approximately 3 to 7 times that of baseline whole blood.28 A fold change of this magnitude would be unlikely to achieve considering the single soft spin utilized by this system. The systems that generate this type of PRP generally concentrate the platelet layer further through additional spin cycles or by apheresis.
Of the different protocols tested in this study, the protocol for group 3 produced PRP with the most consistently increased platelet fold change. Group 3 had the lowest relative centrifugation force and the least time of any of the groups, with 580 X g for 5 minutes. These results may suggest that the manufacturer settings utilize a centrifugation speed that may be higher than optimal. Given the origins of this system in equine patients and the subsequent adoption of the system for canine patients, adjustments to the protocol should be made to tailor the system more narrowly to the specific cellular components of dogs.29,30
The clinical relevance of a lower platelet fold change than the general definition of PRP is unknown, considering previous studies have reported positive outcomes in a model of canine anterior cruciate ligament and meniscal deficiency using this system.31,32 Although the PRP products generated with this system in this study did not concentrate canine platelets to the extent reported for other systems, all groups produced a significantly leukocyte-reduced product (Table 2). These findings suggest that the biologic produced using the ACP double-syringe system may provide greater anti-inflammatory effects than systems that produce leukocyte-rich PRP. Specifically, group 3 had decreased neutrophil and lymphocyte populations but also had statistically significantly concentrated monocytes in addition to platelets. This reduction of selective leukocytes may be an additional benefit of the protocol used in group 3, considering IL-1ra originates from monocytes. IL-1ra acts to mitigate the proinflammatory cytokine, IL-1b, which is linked to the progression of osteoarthritis.33,34
One of the major advantages of this system in small animals is the relatively low volume of starting whole blood. The subjects in this study were all ≥ 17 kg, allowing the blood draws performed to be < 2% of the patients’ total blood volume. However, single weekly blood draws of up to 7.5% of total blood volume (6 mL/kg) have been reported to be safe in dogs.35 With this in mind, this double-syringe system could be used in patients as small as 2.5 kg. The drawback of the low volume of starting whole blood is the potential for low volume of PRP generated. The volume obtained in this study ranged from 0.5 to 7 mL, with no significant difference found among groups. This large volume discrepancy is more likely due to the inherent patient variability and hydration status than due to the differences in group protocols. Often in a clinical setting, multiple joints of a patient may be injected in a single session, and the ideal volume to be injected is yet to be determined. Additionally, the volume of the joint spaces of these small patients will likely necessitate a small volume of therapeutic agent, so as not to overly distend an already inflamed joint. Although the volume an individual blood collection may yield is difficult to predict prior to processing, if a volume deficit arises, it may be mitigated by preparation of a second ACP syringe or with the addition of other intra-articular therapies, such as hyaluronic acid.36
A range of factors affect the quality of PRP generated by a single individual on 1 occasion. However, in clinical settings, the quality of the PRP product is rarely analyzed for cellular composition. The method of analysis used in this study, applied to a clinical setting, would allow practitioners to gain insight into the outcomes achieved following PRP administration.
This study looked to optimize variable parameters within a specific PRP system; this was not a clinical efficacy study. No claims regarding the overall efficacy of PRP therapy in dogs or of the PRP formulations evaluated in this study can be made. Further studies to evaluate the specific cytokine and growth factor concentrations yielded by specific protocols as well as extensive clinical trials are necessary. Due to the inherent patient and user variability associated with biologics, specific factors may influence whether platelets are concentrated in a PRP.
This study demonstrated the importance of evaluating biological samples prior to administration to predict and improve patient outcomes. The future of regenerative medicine in small animals holds incredible promise for treating a plethora of disease processes, yet there is much still to be determined for optimization of these biologics.
Idexx Laboratories provided the CBC analysis for this study.
The authors have nothing to declare.
Alsousou J , Thompson M , Hulley P , Noble A , Willett K . The biology of platelet-rich plasma and its application in trauma and orthopaedic surgery: a review of the literature . J Bone Joint Surg Br . 2009 ; 91 ( 8 ): 987 - 996 . doi: 10.1302/0301-620X.91B8.22546
Garbin LC , Olver CS . Platelet-rich products and their application to osteoarthritis . J Equine Vet Sci . 2020 ; 86 : 102820 . doi: 10.1016/j.jevs.2019.102820
Nguyen RT , Borg-Stein J , McInnis K . Applications of platelet-rich plasma in musculoskeletal and sports medicine: an evidence-based approach . PM R . 2011 ; 3 ( 3 ): 226 - 250 . doi: 10.1016/j.pmrj.2010.11.007
Frisbie DD , Kawcak CE , Werpy NM , Park RD , McIlwraith CW . Clinical, biochemical, and histologic effects of intra-articular administration of autologous conditioned serum in horses with experimentally induced osteoarthritis . Am J Vet Res . 2007 ; 68 ( 3 ): 290 - 296 . doi: 10.2460/ajvr.68.3.290
McCarrel TM , Mall NA , Lee AS , Cole BJ , Butty DC , Fortier LA . Considerations for the use of platelet-rich plasma in orthopedics . Sports Med . 2014 ; 44 ( 8 ): 1025 - 1036 . doi: 10.1007/s40279-014-0195-5
Marx RE . Platelet-rich plasma: evidence to support its use . J Oral Maxillofac Surg . 2004 ; 62 ( 4 ): 489 - 496 . doi: 10.1016/j.joms.2003.12.003
McCarrel TM , Minas T , Fortier LA . Optimization of leukocyte concentration in platelet-rich plasma for the treatment of tendinopathy . J Bone Joint Surg Am . 2012 ; 94 ( 19 ): e143 . doi: 10.2106/JBJS.L.00019
Brossi PM , Moreira JJ , Machado TSL , Baccarin RYA . Platelet-rich plasma in orthopedic therapy: a comparative systematic review of clinical and experimental data in equine and human musculoskeletal lesions . BMC Vet Res . 2015 ; 11 ( 1 ): 98 . doi: 10.1186/s12917-015-0403-z
Sharun K , Jambagi K , Dhama K , Kumar R , Pawde AM , Amarpal . Therapeutic potential of platelet-rich plasma in canine medicine . Arch Razi Inst . 2021 ; 76 ( 4 ): 721 - 730 . doi: 10.22092/ari.2021.355953.1749
King W , Cawood K , Bookmiller M . The use of autologous protein solution (Pro-Stride®) and leukocyte-rich platelet-rich plasma (Restigen®) in canine medicine . Vet Med (Auckl) . 2021 ; 12 : 53 - 65 . doi: 10.2147/VMRR.S286913
Edelmann ML , Mohammed HO , Wakshlag JJ , Ledbetter EC . Clinical trial of adjunctive autologous platelet-rich plasma treatment following diamond-burr debridement for spontaneous chronic corneal epithelial defects in dogs . J Am Vet Med Assoc . 2018 ; 253 ( 8 ): 1012 - 1021 . doi: 10.2460/javma.253.8.1012
Silva RF , Carmona JU , Rezende CMF . Intra-articular injections of autologous platelet concentrates in dogs with surgical reparation of cranial cruciate ligament rupture: a pilot study . Vet Comp Orthop Traumatol . 2013 ; 26 ( 4 ): 285 - 290 . doi: 10.3415/VCOT-12-06-0075
Fahie MA , Ortolano GA , Guercio V , et al. A randomized controlled trial of the efficacy of autologous platelet therapy for the treatment of osteoarthritis in dogs . J Am Vet Med Assoc . 2013 ; 243 ( 9 ): 1291 - 1297 . doi: 10.2460/javma.243.9.1291
Stief M , Gottschalk J , Ionita JC , Einspanier A , Oechtering G , Böttcher P . Concentration of platelets and growth factors in canine autologous conditioned plasma . Vet Comp Orthop Traumatol . 2011 ; 24 ( 2 ): 122 - 125 . doi: 10.3415/VCOT-10-04-0064
Perego R , Spada E , Baggiani L , Martino PA , Proverbio D . Efficacy of a semi automated commercial closed system for autologous leukocyte- and platelet-rich plasma (l-prp) production in dogs: a preliminary study . Animals (Basel) . 2020 ; 10 ( 8 ): 1342 . doi: 10.3390/ani10081342
DeMello V , Chen G , Wakshlag J , Mason D . Evaluation of platelet and leukocyte counts in canine platelet-rich plasma obtained after successive blood collections from the same patient and the effects of freezing on the concentration of growth factors present in it . Front Vet Sci . 2022 ; 9 : 838481 . doi: 10.3389/fvets.2022.838481
Franklin SP , Birdwhistell KE . Assessment of canine Autologous Conditioned PlasmaTM cellular and transforming growth factor-β1 content . Front Vet Sci . 2018 ; 5 : 105 . doi: 10.3389/fvets.2018.00105
Franklin SP , Burke EE , Holmes SP . The effect of platelet-rich plasma on osseous healing in dogs undergoing high tibial osteotomy . PLoS One . 2017 ; 12 ( 5 ): e0177597 . doi: 10.1371/journal.pone.0177597
Farghali HA , AbdElKader NA , AbuBakr HO , et al. Antimicrobial action of autologous platelet-rich plasma on MRSA-infected skin wounds in dogs . Sci Rep . 2019 ; 9 ( 1 ): 12722 . doi: 10.1038/s41598-019-48657-5
Sundman EA , Cole BJ , Fortier LA . Growth factor and catabolic cytokine concentrations are influenced by the cellular composition of platelet-rich plasma . Am J Sports Med . 2011 ; 39 ( 10 ): 2135 - 2140 . doi: 10.1177/0363546511417792
Frye CW , Enders A , Brooks MB , Struble AM , Wakshlag JJ . Assessment of canine autologous platelet-rich plasma produced with a commercial centrifugation and platelet recovery kit . Vet Comp Orthop Traumatol . 2016 ; 29 ( 1 ): 14 - 19 . doi: 10.3415/VCOT-15-03-0046

Ophthalmic Ultrasound de Marcos Carpio I , Sanghani-Kerai A , Solano MA , Blunn G , Jifcovici A , Fitzpatrick N . Clinical cohort study in canine patients, to determine the average platelet and white blood cell number and its correlation with patient’s age, weight, breed and gender: 92 cases (2019-2020) . Vet Sci . 2021 ; 8 ( 11 ): 262 . doi: 10.3390/vetsci8110262